Special Optics brings extensive experience and expertise in advancing quantum computing and atom trapping research by developing custom microscope objectives specifically designed for a range of atomic species, including Rubidium, Strontium, Barium, and Ytterbium.
Expertise in custom microscope objectives
Design and fabrication: Special Optics specialises in designing and fabricating custom microscope objectives that meet the specific needs of researchers working with different atomic species. This includes optimising the optical properties to enhance performance at the specific wavelengths used for laser cooling and trapping.
Wavelength optimisation: Different atomic species absorb and scatter light at specific wavelengths. Our expertise allows us to design optics that maximise efficiency for lasers used with Rubidium, Strontium, Barium, and Ytterbium, ensuring effective atom trapping and manipulation.
High numerical aperture (NA): Custom objectives with high NA are critical for achieving the tight focus needed to trap atoms in optical lattices or tweezers. Special Optics can provide objectives that deliver high resolution and excellent light collection efficiency.
Minimising aberrations: Our optical designs aim to minimise aberrations that could affect the trapping and imaging of cold atoms. High-quality optics ensure that the laser beams remain focused and coherent, which is essential for maintaining the quantum state of trapped atoms.
Integration with experimental setups: Our experience includes collaborating with researchers to integrate custom optics into complex experimental setups, ensuring compatibility with existing equipment like vacuum systems and laser configurations.
Advantages of custom microscope objectives
Enhanced resolution: Custom objectives can provide higher spatial resolution, allowing for better imaging of atomic distributions and dynamics in optical traps. This is essential for examining quantum correlations and entanglement.
Tailored light fields: Custom optics can create specific light patterns (e.g., optical tweezers or lattices) that can trap and manipulate atoms with high precision. This can enable advanced techniques like quantum state engineering.
In-situ measurements: Advanced objectives can facilitate real-time monitoring of cold atoms, enabling the study of dynamical processes and phase transitions in quantum systems.
Integration with quantum computing: In quantum computing, cold atoms can be used as qubits. Custom microscope objectives can help in manipulating these qubits through precise laser pulses, allowing for the implementation of quantum gates and algorithms.
Tailored solutions based on atom species: Choose your wavelength based on your atom. Objective wavelengths can be tailored to support Rubidium, Strontium, Barium and Ytterbium experiments.
Quantum imaging: High-quality imaging systems can enhance the ability to visualise quantum states and their evolution, providing insights that are crucial for both fundamental research and practical quantum computing applications.
Key aspects of cold atom experiments
Cooling techniques: Atoms are typically cooled using laser cooling methods, such as Doppler cooling and evaporative cooling. These techniques help achieve temperatures in the microkelvin range, leading to the formation of Bose-Einstein condensates or Fermi gases.
Trapping methods: Optical traps (using laser light) or magnetic traps are often employed to confine the cold atoms. This spatial confinement is crucial for studying interactions and coherence properties of quantum states.
Measurement and manipulation: Understanding and manipulating the quantum states of atoms requires precise measurement techniques. This is where custom microscope objectives come into play.
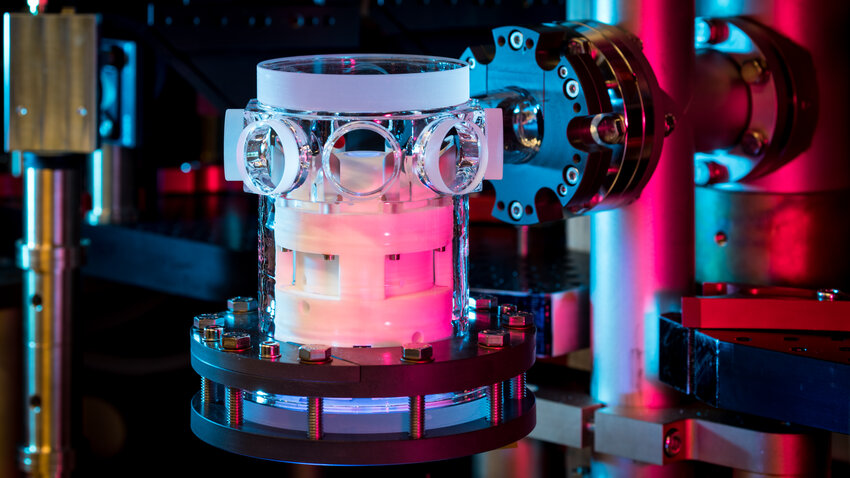
Quartz glass cell with objective under UHV after attachment to the experiment (Credit: Special Optics)
Applications in quantum computing
Quantum gates and circuits: In quantum computing, cold atoms can serve as qubits. Custom microscope objectives enable precise control over the quantum states, allowing researchers to implement quantum gates effectively.
Entanglement and coherence studies: By providing high-resolution imaging and manipulation capabilities, these optics support experiments that investigate quantum entanglement and coherence properties, critical for developing reliable quantum algorithms.
Scalability of qubit systems: As quantum computing aims to scale up the number of qubits, having flexible, high-performance optics is essential for accommodating larger arrays of trapped atoms and complex quantum circuits.
Hybrid quantum systems: The ability to create custom optics that work seamlessly with other quantum systems (e.g., superconducting qubits) allows for innovative hybrid approaches in quantum computing, enhancing the robustness and functionality of the qubits.
Specific support for different atomic species
Rubidium: Often used in laser cooling experiments; objectives are designed for optimal absorption and manipulation using the D-line transitions.
Strontium and Barium: Their unique transition characteristics necessitate specialised optics for precision laser cooling and trapping techniques.
Ytterbium: As a promising candidate for quantum computing due to its long coherence times, custom optics are essential for effectively manipulating its atomic states in various quantum applications.
Future directions
Scalability: Developing optical setups that can easily scale to more atoms or more complex arrangements will be key for future quantum computing architectures.
Hybrid systems: Combining cold atoms with other quantum systems (like superconducting qubits) could lead to hybrid quantum computers that leverage the strengths of different platforms.
Advanced control techniques: The integration of machine learning and adaptive optics could further refine the manipulation of cold atoms, enhancing the precision of quantum operations.
Conclusion
Special Optics plays a crucial role in advancing quantum computing and atom trapping research through our custom microscope objectives. Our ability to tailor optics for specific atomic species and experimental needs enables researchers to explore new frontiers in quantum technology and contributes significantly to the development of scalable quantum computing systems. In summary, the intersection of cold atom experiments and custom microscope objectives presents a rich area of research with the potential to advance our understanding of quantum mechanics and propel the development of robust quantum computing systems.