Choosing an optical filter
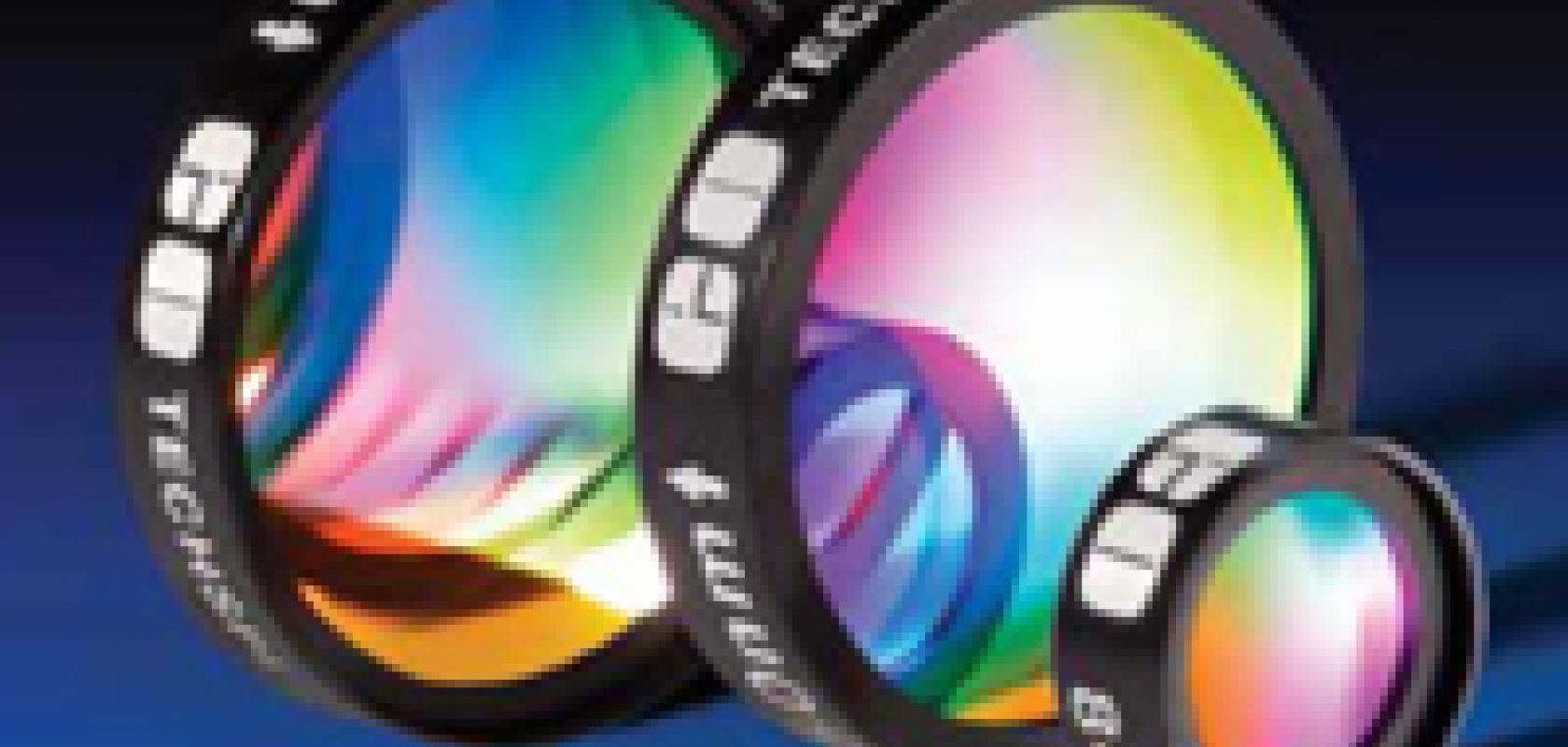
Optical filters can be used to attenuate or enhance an image, transmit or reflect specific wavelengths, and/or split an image into two identical images with controlled brightness levels relative to each other. To understand the importance of choosing the correct optical filter for any application, key terminology, fabrication techniques, and the various types of filters available today need to be considered.
Key optical filter terminology
Before diving into fabrication techniques and the types of optical filters available in the industry today, it is first important to review key terminology associated with them.
Since all filters, independent of how they are manufactured, pass, absorb, and/or reflect some portion of incident light, they share common optical parameters.
Center wavelength
Center Wavelength (CWL) is the midpoint between the wavelengths where transmittance is 50% of the specified minimum transmission, referred to as the Full Width at Half Maximum (FWHM). For interference filters, the peak is typically not at the midpoint wavelength. Refer to Figure 1 for an illustration of CWL and FWHM.
Bandwidth and blocking range
Bandwidth is a wavelength range used to denote a specific part of the spectrum that passes incident energy through a filter. Bandwidth is also referred to as FWHM (Figure 1).
Blocking Range is a wavelength interval used to denote a spectral region of energy that is attenuated by the filter (Figure 2). The degree of its blocking is typically specified in terms of optical density.
Figure 1: Illustration of Center Wavelength and Full Width at Half Maximum
Figure 2: Illustration of Blocking Range
Optical density
Optical Density (OD) describes the blocking specification of a filter and is related to the amount of energy transmitted through it (Equations 1 – 2). A high optical density value indicates very low transmission, and low optical density indicates high transmission. Figure 3 depicts three different optical densities: OD 1.0, OD 1.3 and OD 1.5 that show the higher the OD value, the lower the transmission.
Figure 3: Illustration of Optical Density
Dichroic filter
A Dichroic Filter is a type of filter used to transmit or reflect light, depending on the wavelength; light of a specific wavelength range is transmitted, while light of a different range is reflected or absorbed (Figure 4).
Dichroic filters are commonly used for longpass and shortpass applications.
Figure 4: Illustration of a Dichroic Filter Coating
Cut-on and cut-off wavelength
Cut-On Wavelength is a term used to denote the wavelength at which the transmission increases to 50% throughput in a longpass filter. Cut-on wavelength is indicated by λcut-on in Figure 5.
Cut-Off Wavelength is a term used to denote the wavelength at which the transmission decreases to 50% throughput in a shortpass filter. Cut-off wavelength is indicated by λcut-off in Figure 6.
Figure 5: Illustration of Cut-On Wavelength
Figure 6: Illustration of Cut-Off Wavelength
Optical filter fabrication techniques
Absorptive and dichroic filters
The wide range of optical filters can be broken into two main categories: absorptive and dichroic. The difference between the two does not lie in what they filter, but how they filter. In an absorptive filter, light is blocked based on the absorption properties of the glass substrate used. In other words, light that is blocked does not reflect off the filter; rather, it is absorbed and contained within the filter. In applications where noise in a system from unwanted light is an issue, an absorptive filter is ideal. Absorptive filters also have the added bonus of not being very angle sensitive; light can be incident upon the filter from a wide range of angles and the filter will maintain its transmission and absorption properties.
Conversely, a dichroic filter works by reflecting unwanted wavelengths, while transmitting the desired portion of the spectrum. In some applications, this is a desirable effect because light can be separated by wavelength into two sources. This is achieved by adding a layer, or multiple layers, of material of varying indexes of refraction to exploit the interference nature of light waves. In interference filters, light traveling from a lower index material will reflect off a higher index material; only light of a certain angle and wavelength will constructively interfere with the incoming beam and pass through the material, while all other light will destructively interfere and reflect off the material. (Figure 7).
Unlike absorptive filters, dichroic filters are extremely angle sensitive. When used for any angle(s) outside of their intended design, dichroic filters cannot meet the transmission and wavelength specifications originally indicated. As a rule of thumb, increasing the angle of incidence through a dichroic filter will shift it towards shorter wavelengths (i.e. towards bluer wavelengths); and decreasing the angle will shift it towards longer wavelengths (i.e. towards redder wavelengths).
Figure 7: Deposition of Multiple Layers of Alternating High and Low Index Materials onto a Glass Substrate
Exploring dichroic bandpass filters
Bandpass filters are used in a wide range of industries and can be either dichroic coatings or color substrates. Dichroic bandpass filters are manufactured by two different techniques: traditional and hard sputtered, or hard coated. Both techniques achieve their unique transmission and reflection properties by a deposition of numerous layers of alternating high and low index of fraction materials onto glass substrates. In fact, depending upon the application, there can be more than 100 layers of material deposited per face of a given substrate.
The difference between traditional-coated filters and hard coated filters is the number of substrate layers. In traditional-coated bandpass filters, layers of varying index materials are deposited onto multiple substrates which are then sandwiched together. For example, imagine the illustration in Figure 7 repeated up to and even more than 100 times. This technique leads to a thick filter with reduced transmission. This reduction in transmission is caused by incident light traveling through and being absorbed and/or reflected by numerous substrate layers. Conversely, in hard-sputtered bandpass filters, materials of varying indices are deposited onto only a single substrate (Figure 8). This technique leads to thin filters with high transmission.
Figure 8: Traditional Filter (Left) and Hard Coated Filter (Right)
Types of optical filters
To aid in understanding the similarities and differences between the large variety of optical filters available today, consider ten of the most popular types. The following selection guide contains a brief description as well as sample product images for easy comparison.
Application examples
Example 1: Color match imaging
Monochrome cameras cannot inherently differentiate different colors. However, the addition of a color filter greatly increases the contrast between objects. A good rule of thumb is that a given color filter will lighten objects of the same color, while darkening objects of opposing colors. Consider an example where two red and two green pills are imaged with a monochrome camera. Figures 9a - 9d show actual images of a sample under inspection and various images using color filters. It is clear to see that with no filter (Figure 9b), the monochrome camera cannot distinguish between red and green. It would be impossible to inspect these pills on a factory floor. On the other hand, when a red filter is used (Figure 9c), objects of its opposing color (i.e. the green pills) appear gray due to increased image contrast and can be easily discerned from the red pills. Conversely, when a green filter is used (Figure 9d), the red pills appear gray.
Figure 9a: Contrast Enhancement: Sample under Inspection
Figure 9b: Contrast Enhancement: No Filter
Figure 9c: Contrast Enhancement: Red Filter
Figure 9d: Contrast Enhancement: Green Filter
Example 2: Raman spectroscopy
The results in a Raman spectroscopy application can be greatly improved by the use of a few select filters: laser-line bandpass, rugate notch, or laser-line longpass. To achieve the best results, use filters with bandwidths as narrow as 1.2nm and optical densities of OD 6.0. The laser-line bandpass filter is placed in the optical path between the laser and the sample. This ensures that any external ambient light is blocked and only the laser-line wavelength is passed. After the light is incident on the sample, it is shifted due to Raman scattering and contains many low intensity modes or signals. Therefore, it becomes very important to block the high intensity laser light through the use of a notch filter centered as close as possible to the laser wavelength. If Raman excitation modes occur very close to the laser line, then a laser-line long pass filter can be used as an effective alternative. Figure 10 illustrates a typical Raman spectroscopy setup.
Figure 10: Raman Spectroscopy Setup
Summary
Optical filters are used in a multitude of applications beyond the two aforementioned: color match imaging and Raman spectroscopy. They are encountered in nearly every aspect of the optics, imaging, and photonics industries.
Therefore understanding optical filters’ fabrication techniques, key terminology, and the types of filters available today helps to select the best filter for any setup and to optimize the performance of any final application.
Optical filters can be used to attenuate or enhance an image, transmit or reflect specific wavelengths, and/or split an image into two identical images with controlled brightness levels relative to each other. To understand the importance of choosing the correct optical filter for any application, key terminology, fabrication techniques, and the various types of filters available today need to be considered.
Key optical filter terminology
Before diving into fabrication techniques and the types of optical filters available in the industry today, it is first important to review key terminology associated with them.
Since all filters, independent of how they are manufactured, pass, absorb, and/or reflect some portion of incident light, they share common optical parameters.
Center wavelength
Center Wavelength (CWL) is the midpoint between the wavelengths where transmittance is 50% of the specified minimum transmission, referred to as the Full Width at Half Maximum (FWHM). For interference filters, the peak is typically not at the midpoint wavelength. Refer to Figure 1 for an illustration of CWL and FWHM.
Bandwidth and blocking range
Bandwidth is a wavelength range used to denote a specific part of the spectrum that passes incident energy through a filter. Bandwidth is also referred to as FWHM (Figure 1).
Blocking Range is a wavelength interval used to denote a spectral region of energy that is attenuated by the filter (Figure 2). The degree of its blocking is typically specified in terms of optical density.
Figure 1: Illustration of Center Wavelength and Full Width at Half Maximum
Figure 2: Illustration of Blocking Range
Optical density
Optical Density (OD) describes the blocking specification of a filter and is related to the amount of energy transmitted through it (Equations 1 – 2). A high optical density value indicates very low transmission, and low optical density indicates high transmission. Figure 3 depicts three different optical densities: OD 1.0, OD 1.3 and OD 1.5 that show the higher the OD value, the lower the transmission.
Figure 3: Illustration of Optical Density
Dichroic filter
A Dichroic Filter is a type of filter used to transmit or reflect light, depending on the wavelength; light of a specific wavelength range is transmitted, while light of a different range is reflected or absorbed (Figure 4).
Dichroic filters are commonly used for longpass and shortpass applications.
Figure 4: Illustration of a Dichroic Filter Coating
Cut-on and cut-off wavelength
Cut-On Wavelength is a term used to denote the wavelength at which the transmission increases to 50% throughput in a longpass filter. Cut-on wavelength is indicated by λcut-on in Figure 5.
Cut-Off Wavelength is a term used to denote the wavelength at which the transmission decreases to 50% throughput in a shortpass filter. Cut-off wavelength is indicated by λcut-off in Figure 6.
Figure 5: Illustration of Cut-On Wavelength
Figure 6: Illustration of Cut-Off Wavelength
Optical filter fabrication techniques
Absorptive and dichroic filters
The wide range of optical filters can be broken into two main categories: absorptive and dichroic. The difference between the two does not lie in what they filter, but how they filter. In an absorptive filter, light is blocked based on the absorption properties of the glass substrate used. In other words, light that is blocked does not reflect off the filter; rather, it is absorbed and contained within the filter. In applications where noise in a system from unwanted light is an issue, an absorptive filter is ideal. Absorptive filters also have the added bonus of not being very angle sensitive; light can be incident upon the filter from a wide range of angles and the filter will maintain its transmission and absorption properties.
Conversely, a dichroic filter works by reflecting unwanted wavelengths, while transmitting the desired portion of the spectrum. In some applications, this is a desirable effect because light can be separated by wavelength into two sources. This is achieved by adding a layer, or multiple layers, of material of varying indexes of refraction to exploit the interference nature of light waves. In interference filters, light traveling from a lower index material will reflect off a higher index material; only light of a certain angle and wavelength will constructively interfere with the incoming beam and pass through the material, while all other light will destructively interfere and reflect off the material. (Figure 7).
Unlike absorptive filters, dichroic filters are extremely angle sensitive. When used for any angle(s) outside of their intended design, dichroic filters cannot meet the transmission and wavelength specifications originally indicated. As a rule of thumb, increasing the angle of incidence through a dichroic filter will shift it towards shorter wavelengths (i.e. towards bluer wavelengths); and decreasing the angle will shift it towards longer wavelengths (i.e. towards redder wavelengths).
Figure 7: Deposition of Multiple Layers of Alternating High and Low Index Materials onto a Glass Substrate
Exploring dichroic bandpass filters
Bandpass filters are used in a wide range of industries and can be either dichroic coatings or color substrates. Dichroic bandpass filters are manufactured by two different techniques: traditional and hard sputtered, or hard coated. Both techniques achieve their unique transmission and reflection properties by a deposition of numerous layers of alternating high and low index of fraction materials onto glass substrates. In fact, depending upon the application, there can be more than 100 layers of material deposited per face of a given substrate.
The difference between traditional-coated filters and hard coated filters is the number of substrate layers. In traditional-coated bandpass filters, layers of varying index materials are deposited onto multiple substrates which are then sandwiched together. For example, imagine the illustration in Figure 7 repeated up to and even more than 100 times. This technique leads to a thick filter with reduced transmission. This reduction in transmission is caused by incident light traveling through and being absorbed and/or reflected by numerous substrate layers. Conversely, in hard-sputtered bandpass filters, materials of varying indices are deposited onto only a single substrate (Figure 8). This technique leads to thin filters with high transmission.
Figure 8: Traditional Filter (Left) and Hard Coated Filter (Right)
Types of optical filters
To aid in understanding the similarities and differences between the large variety of optical filters available today, consider ten of the most popular types. The following selection guide contains a brief description as well as sample product images for easy comparison.
Application examples
Example 1: Color match imaging
Monochrome cameras cannot inherently differentiate different colors. However, the addition of a color filter greatly increases the contrast between objects. A good rule of thumb is that a given color filter will lighten objects of the same color, while darkening objects of opposing colors. Consider an example where two red and two green pills are imaged with a monochrome camera. Figures 9a - 9d show actual images of a sample under inspection and various images using color filters. It is clear to see that with no filter (Figure 9b), the monochrome camera cannot distinguish between red and green. It would be impossible to inspect these pills on a factory floor. On the other hand, when a red filter is used (Figure 9c), objects of its opposing color (i.e. the green pills) appear gray due to increased image contrast and can be easily discerned from the red pills. Conversely, when a green filter is used (Figure 9d), the red pills appear gray.
Figure 9a: Contrast Enhancement: Sample under Inspection
Figure 9b: Contrast Enhancement: No Filter
Figure 9c: Contrast Enhancement: Red Filter
Figure 9d: Contrast Enhancement: Green Filter
Example 2: Raman spectroscopy
The results in a Raman spectroscopy application can be greatly improved by the use of a few select filters: laser-line bandpass, rugate notch, or laser-line longpass. To achieve the best results, use filters with bandwidths as narrow as 1.2nm and optical densities of OD 6.0. The laser-line bandpass filter is placed in the optical path between the laser and the sample. This ensures that any external ambient light is blocked and only the laser-line wavelength is passed. After the light is incident on the sample, it is shifted due to Raman scattering and contains many low intensity modes or signals. Therefore, it becomes very important to block the high intensity laser light through the use of a notch filter centered as close as possible to the laser wavelength. If Raman excitation modes occur very close to the laser line, then a laser-line long pass filter can be used as an effective alternative. Figure 10 illustrates a typical Raman spectroscopy setup.
Figure 10: Raman Spectroscopy Setup
Summary
Optical filters are used in a multitude of applications beyond the two aforementioned: color match imaging and Raman spectroscopy. They are encountered in nearly every aspect of the optics, imaging, and photonics industries.
Therefore understanding optical filters’ fabrication techniques, key terminology, and the types of filters available today helps to select the best filter for any setup and to optimize the performance of any final application.